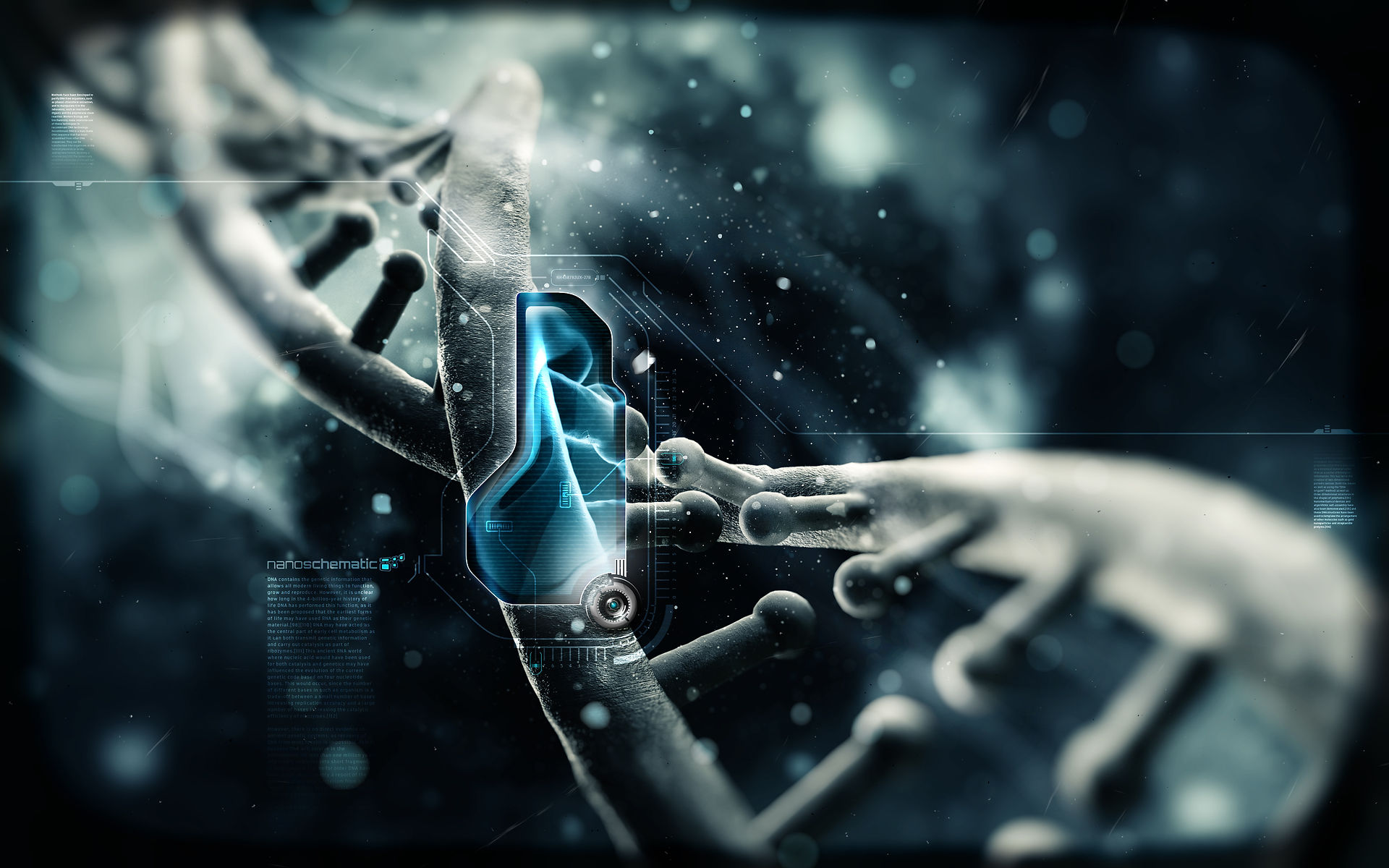
Deoxyribonucleic acid (DNA) and ribonucleic acid (RNA) aptamers are the most common type of aptamers. They are chosen from a pool of random nucleic acid sequences and put through several cycles for optimization in a process called SELEX. These aptamers are single stranded and typically around 15-60 nucleotide bases in length - the longest sequences have been selected at 220 nucleotides.
Upon recognition of their target, aptamers bond by complementary RNA base pairing. This base pairing creates secondary structures such as short helical arms and single stranded loops.
DNA and RNA aptamers are functionally similar but have several differences. DNA aptamers are inherently more stable, cheaper, and easier to produce. RNA also requires reverse transcription, in which RNA must be converted base-for-base into DNA - whereas DNA does not require this extra stage in the SELEX process. DNA and RNA aptamers can also differ in sequence and folding pattern even when selected for the same target.
(Text Citation 5, Text Citation 7, Text Citation 9, Text Citation 14)
How Do Aptamers Work ?
" Most people think of nucleic acids as information holders, not globular things like proteins...what our work proved is that when you do SELEX...you get molecules that, even though they're chemically made out of nucleic acid, are more like proteins than nucleic acids "

Peptide aptamers are small, simple peptides with a single variable loop region tied to a protein on both ends.
Peptide aptamers only bind to their targets with this variable loop region. This contrasts to DNA and RNA aptamers which bind using their entire sequence. Being tied to this loop region reduces the flexibility of peptide aptamers, and thus effectiveness. Peptide aptamers display high specificity properties.
The newest form of aptamers, much is still unknown about these structures. They have found use in the inhibition of a target's ability to interact with proteins.
(Text Citation 5)
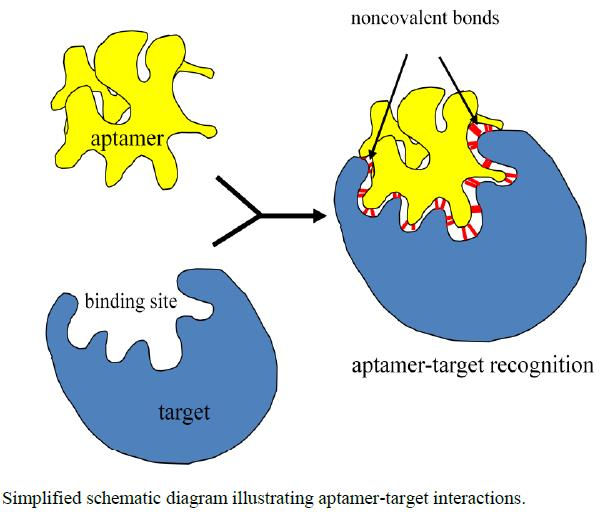
A simplified representation of aptamer-target bonding. As shown, this binding occurs using non-covalent bonds.
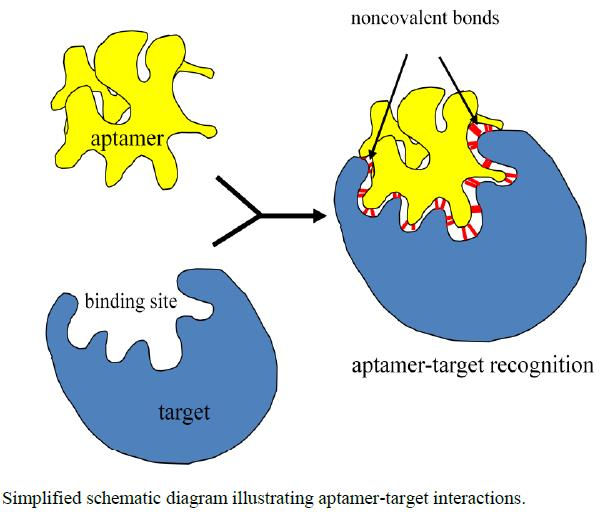
A simplified representation of aptamer-target bonding. As shown, this binding occurs using non-covalent bonds.
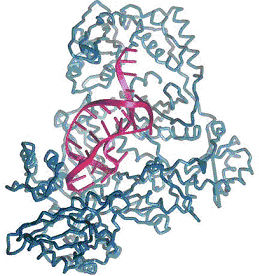
Aptamer (purple structure), fitting and binding to its target (blue)
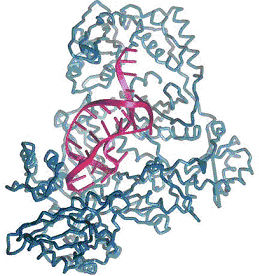
Aptamer (purple structure), fitting and binding to its target (blue)

Three protein recognizing molecules: naturally occurring Antibody (IgG) & T cell Receptor (TCR), and the synthetic peptide aptamer. They have very similar structures, but peptide aptamers are much smaller than the others and its structure does not depend on disulfide bonds - therefore its variable region is more consistent.

Three protein recognizing molecules: naturally occurring Antibody (IgG) & T cell Receptor (TCR), and the synthetic peptide aptamer. They have very similar structures, but peptide aptamers are much smaller than the others and its structure does not depend on disulfide bonds - therefore its variable region is more consistent.
Combination of these secondary structures results in the formation of
tertiary structures that allow aptamers to bind to targets via van der
Waals forces, hydrogen bonding and electrostatic interaction - aligning
with the ways antibodies bind to antigens.
When this tertiary structure forms, the entire aptamer folds into a stable
complex with the target ligand. These are called aptamer-target compl-
exes.
This three-dimensional structure allows aptamers to function like antibodies, which contrasts to traditional thinking which held that DNA/RNA were linear, information holding structures. Aptamer to protein binding ability is in the range of monoclonal antibodies.
- Dr. Larry Gold, Text Citation 4
Peptide Aptamers
DNA & RNA Aptamers
Aptamer Modifications
Aptamer modifications are made to increase aptamer half-lives or for enhanced therapeutic abilities. The primary function of most aptamer modification is to overcome aptamer shortcomings.
A major problem with aptamers is that their small size results in susceptibility to renal filtration and nuclease degradation that cause very short half-lives if left unmodified. In blood, aptamer half-lives are a mere two minutes - a barrier to in vivo, or therapeutic application. However, aptamers can be readily modified to overcome these problems.
Additionally, in certain ways these "disdvantages" can be turned into "advantages," as this natural disposal of aptamers means that additional steps need not be taken to get rid of aptamer-based drugs once introduced into the body. They are also unlikely to be retained inside of the body and cause negative side effects and do not linger in the body for very long.
(Text Citation 2, Text Citation 8, Text Citation 9, Text Citation 62)
Nuclease degradation: Aptamers, as nucleic-acid based molecules, are susceptible to enzymes called nucleases that cleave nucleic acid bonds and can be found throughout the body. This destroys the nucleic acid structure. Aptamer structures can be chemically modified, with modifications to the nucleotide sugars and internucleotide linkages increasing nuclease resistance. "Capping" at the ends of the chains that involve reversing polarity is another strategy, along with inverting nucleotides. Modified nucleotides with functional groups such as fluoro, amino, or O-methyl groups also increase nuclease resistance.
Renal Filtration: Because of their small size, aptamers are subject to filtration by the kidneys. Most aptamers are 5-15 kDa long, or around 15-50 nucleotides. Aptamers for commercial applications are often shortened as much as possible to reduce manufacturing costs. The cutoff for renal filtration is around 30-50 kDa, so conjugation to a polymer with high molecular mass called polyethylene glycol (PEG) can make aptamers resistant to renal filtration.
Conjugation: Aptamers can be conjugated, or joined with, additional units that result in new properties for the aptamer. For example, a non-nucleic acid unit can be joined to an aptamer to allow for additional bonds to be formed between the target and the aptamer that would not be possible without the additional unit.
Additionally, aptamers can be conjugated to drugs, functioning as drug-delivery systems. For example, aptamers have been conjugated with chemotherapy drugs, and because they seek out their targets with high affinity and specificity, they target and bind to only cancerous cells and leave healthy tissue untouched - ensuring their drug cargo is delivered correctly.
Other examples of this concept include conjugation to toxins and
small interfering RNAs (siRNAs). siRNAs silence gene expression,
but their usefulness has been limited by delivery problems -
aptamers provide an ideal solution when conjugated.
(Text Citation 2, Text Citation 8, Text Citation 9, Text Citation 62)
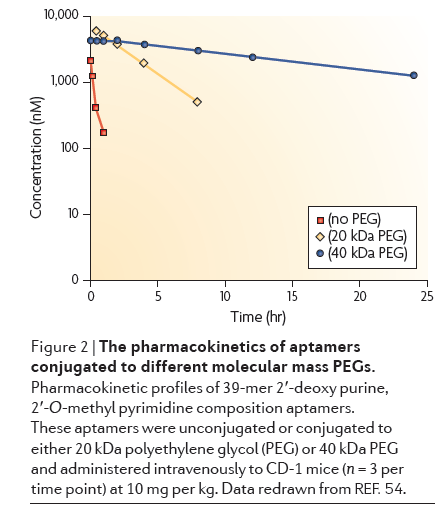
Click to expand: Chart showing different sized PEG effect on half-life
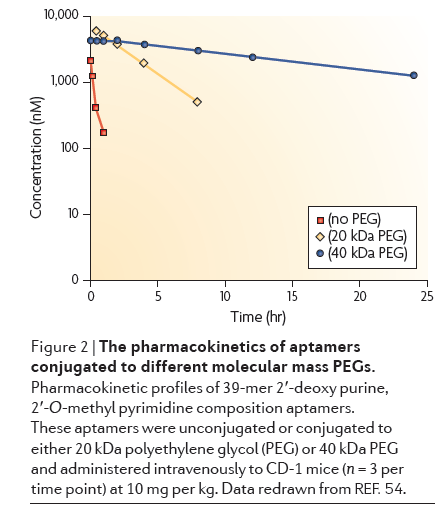
Click to expand: Chart showing different sized PEG effect on half-life
There are three types of aptamers: DNA, RNA, and peptide aptamers. All have very similar properties but are distinctly unique.
It is theoretically possible for aptamers to be used against any molecular
target - aptamers have been selected against small molecules, toxins,
peptides, proteins, viruses, bacteria, and even whole cells.
Aptamers bind with high specificity and affinity - meaning that they
discriminate for their specific targets with high precision - once they have
determined their target, they then bind to it with a strong bond.
Because they structurally conform to bind to their targets, this gives
aptamers a wider range of possible targets when compared with
antibodies, which require antigens and epitopes along with an immune response for their targets.
(Text Citation 4, Text Citation 5)
Page Contents
tid
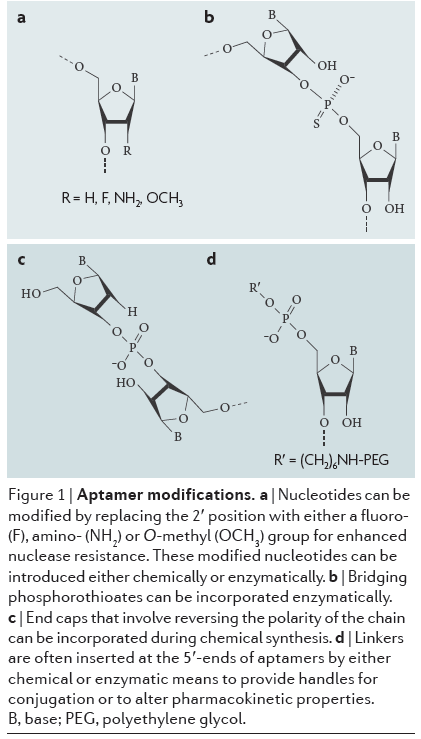
Click to expand: Aptamers with various chemical modifications to increase nuclease resistance.
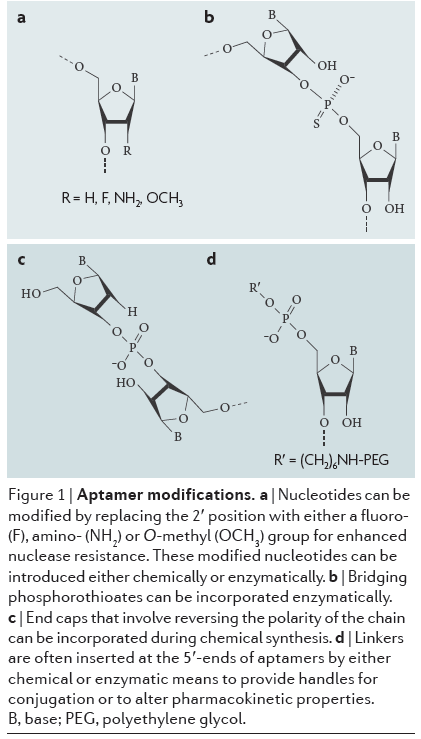
Click to expand: Aptamers with various chemical modifications to increase nuclease resistance.

Text Citation 9 - RNA aptamer binds to Vitamin B12

Text Citation 9 - RNA aptamer binds to Vitamin B12
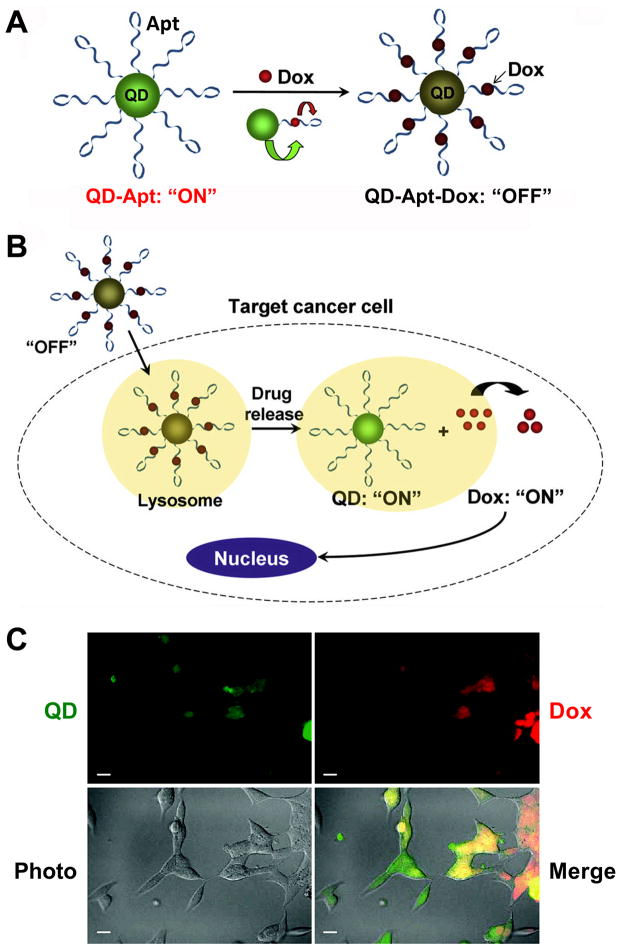
See description at left.
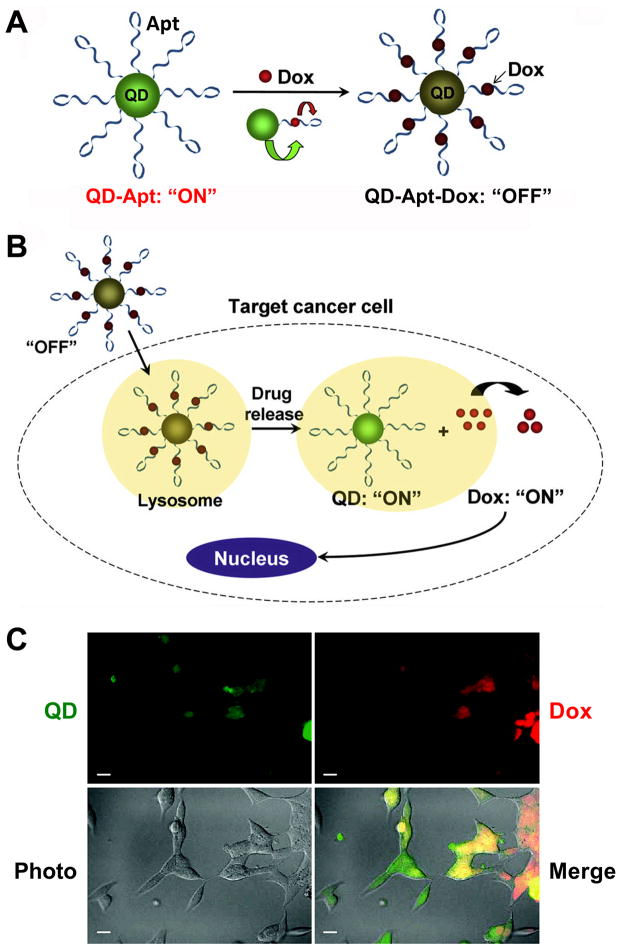
See description at left.
Image at Right: A QD-Apt-Dox conjugate. (Dox = Doxorubicin, a chemotherapy drug) - Scale bar: 20 μm.
A. QD-Apt-Dox is initially “off” as the fluorescence of QD is transferred to Dox and the fluorescence of Dox is quenched by the aptamer, both by fluorescence resonance energy transfer.
B. Once QD-Apt-Dox is inside cancer cells, Dox is gradually released from the conjugate and the fluorescence of QD is recovered.
C. Microscopy images of PSMA-positive cells after incubation with QD-Apt-Dox. QD and Dox are shown in green and red, respectively.
• DNA & RNA Aptamers
• Peptide Aptamers
• Aptamer Modifications
• Nuclease Degradation
• Renal Filtration
• Conjugation